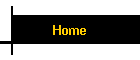
| |

Muscle
and Blood
How does the body work?
This question about the general nature of the life process is as old as human
thought. Both philosophers and physicians have speculated and written about the
mechanism of life and the special functions of body organs. Ancient man believed
that the heart was the home of the soul. Fortunetellers used the livers of
animals to try to predict the future. From these crude beginnings arose the
science of physiology.
Physiology appeared a gruesome and reprehensible business-‑certainly not a
gentleman's pursuit. A first‑century Roman scholar, Celsus, wrote a horrifying
description of the activities of Alexandrian scientists: "They laid open men
whilst alive, criminals received out of prison from the kings, and whilst these
were still breathing, observed parts which beforehand nature had concealed,
their position, colour, shape, size, arrangement, hardness, softness,
smoothness, relation, processes, and depressions of each, and whether any part
is inserted into or is received into another." Celsus deplored these
experiments. "I believe that medicine should be rational. . . . But to open the
bodies of men still alive is as cruel as it is needless." Further advances in
physiology came from the dissection of dead animals and live ones.
The busiest dissector and vivisector in Rome was the second‑century physician
Galen. Though he never cut up ants, gnats, fleas, "and other minuscule
creatures," Galen did manage to dissect the remainder of the animal kingdom:
apes, horses, asses, mules, cows, camels, sheep, Eons, wolves, dogs, lynxes,
stags, bears, weasels, mice, snakes, a variety of fish and birds, and several
elephants.
Galen limited dissection of live animals to pigs or goats, which he strapped to
a board. Although he obtained much anatomical information from the barbary ape,
he usually would not vivisect this little tailless creature, and recommended to
other scientists that they "leave live apes alone." Perhaps the ape's facial
expressions and cries while being cut up were human enough to be disturbing.
From his animal studies Galen managed to learn a few fundamental facts about
how the body works. In one experiment, he cut the recurrent laryngeal nerve of a
live pig and found that the animal could no longer squeal, thus identifying the
origin of the voice and part of its control mechanism. Even more elegant was his
identification of the optic nerve as being responsible for vision:
"When you have divided the frontal bone . . . you will be met by two nerves that
go to the eye. If you divide the larger of the two, then the visual sense of the
animal will be impaired. . . . But that the animal can no longer see . . . you
can only appraise by deduction, from the fact that you find that it does not
blink with its eye at anything which you bring near it, pretending to be about
to stab home with it."
Galen also made the first correct observations of the functions of the spinal
cord and the kidney. He noted that if the cord is cut "behind the first thoracic
rib, then that damages the hand of the ape. And should the cut follow a line
behind the second thoracic rib, then that does not damage the arm, except that
the skin of the axillary cavity and upper arm become deprived of sensibility."
In another experiment, the ureter of a living animal was tied, proving that
urine is produced in the kidney.
Galen should have stopped here, but he didn't. Instead, he tried to use what he
had found and what he could contrive to explain one of the oldest of all
mysteries: what the heart does, and why it is necessary to breathe, He failed,
and fifteen centuries elapsed before this interaction of muscle and blood was
understood-‑the first triumph of the science of healing.
Of Pumps and Valves
A single scientist, William Harvey, made the discovery of the circulation of the
blood. Even today, more than three hundred years later, Harvey's finding is
considered the most important advance in all of physiology, on a par with Isaac
Newton's discovery of universal gravitation in physics. Our knowledge of the
spread of infections and of the cause of many diseases depends on comprehension
of blood circulation; without this comprehension, most of medicine as we know it
today would not exist and doctors would be little more than witch doctors.
The recognition that the heart and blood are necessary for life is a primitive
one. An association between cold, pallor, bloodlessness, and death has been
common knowledge since antiquity. Vampires were believed to kill a victim by
sucking out his blood, and the bloodiest of all wounds, a heart wound, was known
to be invariably fatal.
The first detailed anatomic study of the heart was made around 400 B.C. and is
included in a collection of ancient Greek medical treatises known as the
Hippocratic corpus. The author, perhaps a Greek from Sicily, dissected the
mammalian heart with exceptional skill, and though he saw it as having only two
chambers rather than the four we know today, he discovered within it two
interesting sets of membranes. There are two great vessels, he wrote, and "at
the entrance of each are arranged three membranes, rounded at their
extremities, in the shape of a half circle; and when they come together, it is
marvelous to see how they close their orifices. . . . And if someone . . . takes
the heart after death, and the membranes are spread out and made to lean against
each other, water poured in will not penetrate into the heart, nor will air
blown in; and this especially on the left; for that side has been constructed
more precisely, as it should be, since the intelligence of man lies in the left
cavity."
The membranes this unknown anatomist describes are the aortic and pulmonary
valves. He obviously interprets them as static safety devices to prevent a messy
mixing of blood and intelligence, testing his conclusion by pouring water into
the stumps of the aorta and pulmonary artery to check whether the valves close
properly; this is now routine in modern pathology. But he goes further by
blowing into the severed vessels, a procedure not taught in pathology
residencies today.
About 270 B.C., an Alexandrian physician, Erasistratos, made the next
contribution to cardiac physiology. Erasistratos discovered that the heart is
not a static reservoir. It is a pump, with the right and left portions
subdivided into upper and lower chambers (atrium and ventricle) separated by the
bicuspid and tricuspid valves. These valves are made respectively of two and
three roughly triangular flaps, anchored to the inner surface of the heart by
cords. When the heart contracts, the valve flaps are pressed together to
prevent blood from returning to the upper chambers. Erasistratos concluded
correctly from his observations that the heart must receive blood from the veins
and pump it out through the arteries.
Where the blood came from and went remained a mystery. Veins and arteries were
seen as sets of independent, dead‑end canals. Blood and air were supposed to
seep slowly toward the periphery, where they were used up. The lungs were
considered to be chiefly for the purpose of cooling the blood. Galen recognized
that the arteries contained blood, and not air as had been believed, but he
still managed to explain the nature of the pulse beat incorrectly, and his
faulty explanation was perpetuated by his writings for nearly fifteen hundred
years.
Andreas Vesalius, a professor of surgery and anatomy at the University of Padua,
finally published a correct description of the anatomy of the heart in 1543. In
his book De Humani Corporis Fabrica, Vesalius showed that Galen had made two
serious mistakes. The ascending vena cava, which Galen had originating in the
liver, was demonstrated to be one of the two great veins which bring blood from
the body back to the right side of the heart. Perhaps Galen had confused the
vena cava with the hepatic vein, which does arise from the liver.
Galen's description of the septum dividing the right side of the heart from the
left was also incorrect, for he somehow believed that it had openings connecting
the two ventricles. How Galen could have arrived at this conclusion remains
uncertain. Some abnormal hearts do have a septal opening, and surgeons today
correct this problem by sewing a dacron patch over it. Galen might have noted
such an orifice in one or two animal hearts and regarded it as the norm.
Vesalius, however, was able to show conclusively that the cardiac septum
contains no openings.
For rectifying these and other mistakes Galen had made, Vesalius was showered
with a torrent of abuse by his contemporaries. Jacobus Sylvius, a former
teacher, turned against his pupil angrily, calling Vesalius a madman. A former
assistant, Realdus Colombo, sought to discredit and deride his teacher. Vesalius
was sensitive to such criticism; out of rage and disappointment he was reported
to have burned notes being prepared for another publication.
Within a few years the findings of Vesalius had been verified. And when combined
with the work of two other men, Servetus and Colombo, they formed the anatomic
basis for understanding blood circulation.
Miguel Servetus was a fellow student with Vesalius in Paris. At some period
during his life as a physician he began to study the lungs and realized that
blood filtered through them, mixed with air, changed color, and entered the left
side of the heart. Servetus turned his attention to religion and wrote a book
critical of the holy Trinity. Persecuted, he fled to Geneva, hoping to find
protection with another fellow student from Paris, the reformer John Calvin.
But Calvin was not sympathetic and had him tried and condemned for heresy.
Servetus was burned at the stake in 1553, supposedly with all copies of his
works. Three did escape the flames, though we are uncertain as to whether his
anatomical ideas were immediately appreciated.
Another anatomist working at the same time as Servetus, Realdus Colombo, also
observed the circulation of blood through the lungs and the mixing there with
air, and in addition noted the simultaneous beat of the two ventricles of the
heart. Colombo published his findings in a book that appeared in the year of his
death, 1559.
Now the anatomical information necessary for understanding the circulation of
the blood was available, and the parallels with Newton's accomplishment, which
occurred in the same century as William Harvey's, can be seen. Newton was able
to take Johannes Kepler's laws of planetary motion, combine them with Galileo's
laws of the motion of a falling body, and from these derive the notion of
universal gravitation. Harvey was able to take the isolated facts known about
the cardiovascular system and use them to explain just how this system works. In
doing so, he laid the scientific basis for all of modern medicine.
William Harvey was born at Folkestone, in England, April 1, 1578. He was the
eldest child in the large family of Thomas Harvey, a prosperous merchant and
civic official. Several brothers became successful merchants, but William's
scholarly nature destined him early in life for one of the professions. The
scholarliness was still in evidence years later: While accompanying King Charles
I and taking care of the princes during the battle of Edgehill, Harvey is
described as sitting at the outskirts of the fight under a hedge reading a book.
Harvey was sent to King's School at Canterbury and then to Caius College at
Cambridge, where he received the Bachelor of Arts degree in 1597. Shortly
afterward, he enrolled at the medical school of the University of Padua. Here,
where Vesalius had written his book, another anatomist, Fabricius of
Aquapendente, was at the peak of a distinguished career, lecturing in the
windowless, six-tiered, oval amphitheater he had had specially designed for
teaching anatomy. By candlelight, Harvey and several hundred other students
stood and watched as the master dissected, disposing quickly of the unpreserved
corpses to prevent their reek from overpowering everyone in the closed room.
Years later, Harvey was to credit Fabricius with the discovery that first
inspired the young anatomy student to consider the circulation of the blood.
This was the identification of the venous valves.
Actually, Johannes Baptista Cannanus, a contemporary of Vesalius, had observed
the little venous valves long before Fabricius. But the first meticulous
descriptions were not made until Fabricius published his book devoted to the
subject, De Venarum Ostiolis. In this work, the structure, position, and
distribution of the little valves are carefully noted and illustrated by fairly
good drawings. Fabricius also clearly recognized that the valves offer
opposition to the flow of blood from the heart towards the periphery, but failed
to comprehend their true function of preventing retrograde flow, believing
instead that they merely prevented too much blood from being heaped in one
place. Harvey did not make this mistake.
After receiving the Doctor of Medicine degree from the University of Padua in
1602, Harvey returned to England, and in the same year was awarded another
doctoral degree in medicine from Cambridge. He then settled in London to
practice medicine, and was admitted as a candidate to the College of Physicians
in 1604. In November of that year he married Elizabeth Browne, daughter of
Lancelot Browne, former first physician to Queen Elizabeth.
What sort of man was this rising young doctor? Like Shakespeare, his
contemporary, Harvey left us his works but not very much about himself. Most of
our knowledge about his character derives from a librarian and biographer, John
Aubrey. Harvey, wrote Aubrey, was a very short man with a "little eie, round,
very black, full of spirit." He was temperamental and somewhat eccentric. As a
young man he wore a dagger, in the fashion of the day, but was prone to draw it
upon the slightest provocation. In his later years he liked to be in the dark
because, he said, he could think better, and had underground caves constructed
at his house in Surrey for meditation.
We do not know when Harvey began to form his notion of the circulation of the
blood. His first musings on the subject appeared in a series of anatomical
lectures that he delivered in 1616. His ninety-eight-page set of notes still
exists, and besides his genius these demonstrate that Harvey was a copious
scribbler. He wrote hastily and almost illegibly in a mixture of Latin and
English, and was a careless speller. In one place in his notes the word "piggg"
appears, with a rather large number of g's even for seventeenth‑century English.
His writings tell us that Harvey was initially quite overwhelmed by the enormous
complexity of the cardiovascular system:
"When I first gave my mind to vivisections, as a means of discovering the
motions and uses of the heart, and sought to discover these from actual
inspection, and not from the writings of others, I found the task so truly
arduous, so full of difficulties, that I was almost tempted to think . . . that
the motion of the heart was only to be comprehended by God. For I could neither
rightly perceive at first when the systole [contraction] and when the diastole
[relaxation] took place, nor when and where dilatation and contraction occurred,
by reason of the rapidity of the motion, which in many animals is accomplished
in the twinkling of an eye, coming and going like a flash of lightning; so that
the systole presented itself to me now from this point, now from that; the
diastole the same; and then everything was reversed, the motions occurring, as
it seemed, variously and confusedly together. My mind was therefore greatly
unsettled, nor did I know what I should myself conclude, nor what to believe
from others. . . ."
Harvey solved the problem with a set of ingenious experiments. Though in some
his calculations were incorrect, he managed to come to the right conclusions.
There can be no doubt that Harvey had realized what he was going to find before
he formally began to look for it.
The first step was to prove that the amount of blood transmitted from the veins
to the arteries is so copious that all the blood in the body must pass through
the heart in a short time. Physicians since Galen had erroneously believed that
blood was constantly being produced from food consumed. To accomplish his proof,
Harvey attempted to measure the amount of blood that the heart ejects with each
beat and to establish the pulse rate.
Even today, the measurement of cardiac output is a complex and difficult
procedure, and there are wide variations in results obtained by various methods.
It is not surprising, then, that Harvey's measurements were not correct; but he
arrived at a ridiculous figure, far below the lowest estimate used today. No
doubt he was not a skilled experimenter.
Harvey derived his results by measuring the volume of the left ventricle in one
cadaver, then multiplying this figure by the pulse rate. But, in the first
place, he measured the volume incorrectly, and then he made an enormous error
by using a pulse rate of thirty‑three beats per minute, about half the actual
average rate. The final figure he obtained for cardiac output is less than one
thirty‑sixth of the lowest value accepted today. Nonetheless, Harvey had proved
his point, because even by his calculations the output of the heart in thirty
minutes far exceeded the total weight of blood in the body. Obviously Galen had
been quite wrong in believing that the amount of food a man eats could produce
blood continuously in any such volume.
The second step of Harvey's proof was to demonstrate that the amount of blood
going to the extremities is much more than is needed for the nutrition of the
body. Here he used no specific measurements and argued instead largely by
inference. In doing so, he made the important point that the blood must pass
from the arteries to the veins in the extremities. This was ingeniously
demonstrated by employing a bandage in such a way as to stop the flow in the
veins of a man's arm while leaving the arteries open. As a result, the veins
swelled but not the arteries. When the pressure was increased sufficiently to
cut off arterial circulation as well, the veins did not swell. From his
observations, Harvey reasoned correctly that the blood entered the extremities
through the arteries and passed somehow to the veins. He looked for the
channels of connection but, lacking a microscope, failed to find them. In 1661,
four years after Harvey's death, Marcello Malpighi, using a crude microscope,
located these tiny channels we now call capillaries in the lung of a frog.
The third step was to prove that blood in the veins flows toward the heart and
not away from it, as Galen had believed. Harvey demonstrated this in an
elegantly simple manner: He pressed one finger on a vein in a man's arm and
moved the finger along the vein from below one valve to above the next. The
blood thus pushed up the vein did not return to the emptied section. This
established beyond doubt that the valves were one‑way devices, thus destroying
Galen's old theory that blood moved back and forth in the venous system like the
ebb and flow of the tide.
In 1628 Harvey published his experiments in a little book entitled Exercitatio
Anatomica de Motu Cordis et Sanguinis in Animalibus "An Anatomical Treatise on
the Movement of the Heart and Blood in Animals" or De Motu Cordis. Here are his
conclusions, soon destined to change the course of all medical thought:
"Since all things, both argument and ocular demonstration, show that the blood
passes through the lungs and heart by force of the ventricles, and is sent for
distribution to all parts of the body, where it makes its way into the veins and
pores of the flesh, and then flows by the veins from the circumference on every
side to the centre from the lesser to the greater veins, and is by them finally
discharged into the vena cava and right auricle of the heart, and this in such
quantity or in such afflux and reflux, thither by the arteries, hither by the
veins, as cannot possibly be supplied by the ingesta, and is much greater than
can be required for mere purposes of nutrition; it is absolutely necessary to
conclude that the blood in the animal body is impelled in a circle, and is in a
state of ceaseless movement; that this is the act or function which the heart
performs by means of its pulse, and that it is the sole and only end of the
movement and contraction of the heart."
Harvey probably never had any notion of the far‑reaching consequences his
discovery would have. Nothing else can explain the lack of care devoted to De
Motu Cordis. The manuscript was sent to an obscure German printer, Wilhelm
Fitzer of Frankfurt‑am‑Main, and was produced on thin, cheap paper that quickly
deteriorated. The finished work teemed with typographical errors, suggesting
that Harvey did not even take the trouble to read proof. Except for the title
page and two plates borrowed from Fabricius, there were no illustrations. But
there was no lack of controversy.
The loudest critics were Jean Riolan, an anatomist on the faculty of the
University of Paris, and his English student James Primrose. Riolan managed to
induce his university to prohibit the teaching of Harvey's doctrine. Primrose,
who had just been certified to practice medicine in England the year before with
Harvey as one of his examiners, locked himself in a room for two weeks and
produced quite a large book that refuted De Motu Cordis by rehashing all the old
ideas. Harvey began to be referred to in some circles as "Circulator" ‑-in the
Latin sense of the word, which means quack. He preserved a stoic silence and in
the end lived to see his work vindicated. In the meantime, his professional
standing was quite unaffected because of his royal patrons.
This patronage began in 1609, when his brother John Harvey, who had obtained
employment in the king's household, influenced James I to recommend William for
an appointment at St. Bartholomew's Hospital as assistant physician. When the
physician died in the summer of that year, Harvey succeeded him. The hospital at
that time had about two hundred beds for patients in twelve wards, and the new
physician's duties consisted of attending in the hall of the hospital for at
least one day a week throughout the year and prescribing for treatment at any
other time when specially needed. The physician was usually expected to live
within the hospital grounds, but the rule was waived for Harvey since he lived
not far away. He received an annual salary of twenty‑five pounds, with two
pounds extra for livery and a further eight pounds since he did not use the
official residence. Three surgeons and an apothecary in charge of the dispensary
formed the remainder of the staff.
In his free time, Harvey developed a large private practice, attending many of
the most distinguished citizens, including Sir Francis Bacon and, after about
1618, King James himself as physician extraordinary. Though advanced in his
physiologic views, Harvey was quite conservative in the remedies he prescribed
for his patients; considering the wealth of worthless drugs and primitive state
of medical therapeutics, such conservatism was probably best.
In 1625 King James fell ill for the last time, and Harvey led the team of
doctors in attendance. After the king's death, a rumor quickly spread that his
favorite, the Duke of Buckingham, had hastened the fatal outcome by applying
remedies not approved by the doctors. As suspicion grew, the duke was actually
accused of having poisoned the king, and in 1626 an inquiry was ordered by
Parliament. Harvey was the most important witness of several who contributed to
saving the duke's neck.
What may be called the best years of Harvey's life began with the ascent of the
new king, Charles I. The appointment as physician extraordinary was continued,
and Harvey received a special award for the care he had given the previous
monarch. The new king and his physician quickly became the closest of friends,
with the king always ready to help in furthering the biologic research. Harvey,
in return, delighted in showing Charles anything of scientific interest. On one
occasion a courtier received a severe chest injury that exposed his heart.
Harvey was called to attend and he summoned the king, who was permitted to stick
his fingers into the wound to feel the beating heart. If infection subsequently
developed, at least the organisms were royal.
Harvey might have lived the rest of his days as a close confidant of the king
with an excellent position but for the Civil War in England. Like many
scientists before and since, Harvey had little interest in politics, but he was
soon to learn how a changed political climate could so sour his life as to make
it barely tolerable.
Parliament had become quite restive under Elizabeth, though deferring to her as
an aging woman and a national symbol. Neither James I nor his son Charles was to
have this good fortune. Parliament would not grant either of these rulers
adequate revenue, because it distrusted them both. Many members were Puritans,
dissatisfied with the organization and doctrine of the Church of England. And
Parliament was organized so that it could make resistance effective.
In 1629 the king and Parliament came to a deadlock. Charles attempted to rule
without Parliament, which could legally meet only at the royal summons. The
Scots were the first to rebel, rioting in Edinburgh in 1637 against attempts to
impose the Anglican religion in Scotland. To raise funds to put down the
Scottish rebellion, Charles convoked the English Parliament in 1640, for the
first time in eleven years. When it proved hostile to him, he dissolved it and
called for new elections. The same men were returned. The resulting body, since
it sat theoretically for the next twenty years without new elections, is known
historically as the Long Parliament.
The Long Parliament, far from assisting the king against the Scots used the
Scottish rebellion as a means of pressing its own demands. These were
revolutionary from the outset. Parliament insisted that the chief royal
advisers‑-Harvey was one-‑be not merely removed but impeached and put to death.
In 1642 Parliament and king came to open war.
At the start of this civil war, Harvey was with the king. When Charles later
established his headquarters at Oxford, Harvey remained with him, and in 1645
was made warden of Merton College. Here he resumed the work on embryology that
he had begun years earlier with deer embryos, which was to result in the
publication of his second book, De Generatione Animalium; or, Anatomical
Excitations, Concerning the Generation of Living Creatures. But while he
puttered with eggs and yolks, a powerful leader was coming to the fore.
This was a hitherto unknown gentleman named Oliver Cromwell, a devout Puritan
and member of the parliamentary forces called Roundheads, from the close
haircuts they wore. Cromwell was able to organize a new and more effective
military force, the Ironsides, in which extreme Protestant exaltation provided
the basis for morale, discipline, and the will to fight. Gradually these men
were able to crush the Royalist opposition.
Cromwell concluded that the defeated King Charles could not be trusted, that
"ungodly" persons of all kinds put their hopes in him (what later ages would
call counterrevolution), and that be must be put to death. Since Parliament
resisted, Cromwell, with the support of the army, broke Parliament up.
When the defeated Charles fled from Oxford to surrender himself to the Scots,
Harvey joined him for a time at Newcastle but was forced to leave the king when
he was handed over to the parliamentary army, and was not allowed to go to him
when he was imprisoned in the Isle of Wight. Charles' execution in 1649 left
Harvey a broken and unhappy man, though even before this he had begun to suffer
the consequences of having supported the wrong faction.
In 1643 he had been stripped of the post at St. Bartholomew's Hospital, one he
had occupied for thirty‑four years. His professional reputation was gradually
eroded, and in his last years under Cromwell's Protectorate he was regarded as
a political "delinquent," being forced to spend much of his time lodging in one
or another of his brothers' houses outside London. During the Civil War his
house was sacked and parliamentary soldiers destroyed most of his papers. The
once‑prominent physician was reduced at the end almost to incompetence in the
eyes of his patients, as one, the Lady Anne Conway, describes in a letter to a
friend:
"I heare that you have a great good opinion of Dr, Harvey. I thinke you do well
. . .: he is a most excellent anatomist, and I conceive that to be his
Masterpiece, which knowledge is many times of great use in consultations, but in
the practicke parte of Physicke I conceive him to be too mutch, many times
governed by his Phantasy, the excellency and strength whereof did produce his
two workes to the world. . . .
"I grieve much Dearest that you are not yet out of Dr. Harvey's hands. . . .
"When once I have ended my tryalles of Dr. Harvey which I thinke will be very
shortly. He is very ill himselfe of the gowte almost continually, and that must
needs indispose him to the mindings of such things as relates not to his owne
perticuler (yet he pretends very much to study and lay my case to heart)."
Another colleague put the matter more succinctly: "I know several practitioners
that would not have given threepence for one of his bills."
Deeply despondent, racked with pain by gout and kidney stones, Harvey at age
eighty was forced to move once again, this time to his brother Eliab Harvey's
house at Roehampton. There he awoke one morning, partially paralyzed and unable
to speak, and shortly after, on June 3, 1657, he died.
The Flower of English Medicine
At the time of Harvey's death his discovery was still only of theoretical
interest. What, after all, could a physician offer a patient whose heart was not
contracting properly? This question probably never even occurred to Harvey,
because no one at the time recognized the relationship between heart disease and
dropsy, a condition in which the tissues and cavities of the body fill up with
fluid, frequently on account of a weak heart.
The supreme discovery in the field of cardiology was the identification of
digitalis, a drug so effective for treating a weakened heart muscle that doctors
have found no better substitute in two hundred years. The discoverer was an
English physician, William Withering.
For centuries the common form of dropsy, now called cardiac edema, was one of
the most frequent causes of death, and an unpleasant death it was. Dr. Samuel
Johnson, the eighteenth‑century lexicographer, died of dropsy after suffering
intensely, his legs becoming so painfully bloated that his physicians vainly
tried to let the fluid out by making large knife incisions.
Yet since antiquity a few rather bizarre and unreliable concoctions sometimes
helped a patient with dropsy. The dried bulb of the squill, a plant of the lily
family native to the Mediterranean area, was known as a medicine to the ancient
Egyptians and is mentioned in the Ebers Papyrus, written around 1500 B.C. The
Romans used squill to treat dropsy, to strengthen the heart, to induce vomiting,
and—ominously-‑to poison rats, for the effective ingredient in squill, called a
cardiac glycoside, will accomplish all these functions as the dose is increased.
Strophanthus, the seeds of a genus of African shrubs and woody vines used to
make arrow poison, contains another cardiac glycoside and was also introduced
into medicine. The Chinese have used the dried skin of the common toad for
centuries as a drug. Called ch'an su, it was highly recommended for toothache
and bleeding of the gums, as well as dropsy. We now know that ch'an su contains
epinephrine, an arterial constrictor, combined with a cardiac glycoside. The
beneficial effects of ch'an su were also known to the peasants of Western
Europe, who had used powdered toad skins medicinally for centuries.
These same peasants favored one folk remedy above all others. This medicine came
from a tall plant with long pointed leaves and lovely, delicate purple flowers
shaped like bells. For centuries it had grown wild through most of Europe, and
its dried, powdered leaves were known to bring relief to many dropsy sufferers.
This plant was Digitalis purpurea, the purple foxglove.[1]
We know that digitalis had been used since at least the thirteenth century by
Welsh physicians, for they mentioned it in their writings as menygellydon, which
means "elves gloves." The word foxglove is of uncertain origin, but some
etymologists believe it is derived from the name of an ancient musical
instrument that consisted of bells hung from an arched support; very likely the
little purple bell‑shaped flowers hanging from the foxglove stalk looked
similar. Even the Norwegian word for foxglove means "fox music."
In 1542 the, first accurate scientific description of foxglove was given by a
German botanist, Leonhard Fuchs. He named the plant genus Digitalis, from the
Latin digitus, meaning "finger"; but why he chose this word is uncertain.
Perhaps the German name for the plant, Fingerhut, meaning "thimble," was the
origin. More likely, the tall, fingerlike vertical stalk from which the foxglove
flowers hang suggested the word.
Fuchs did more than name Digitalis purpurea. In his 1542 history of plants, he
categorized digitalis as being of value in inducing vomiting and "in its action
to thin, to dry up, to purge, and to free of obstructions." John Gerard
confirmed one of these observations in 1597, noting that he had used foxglove to
induce vomiting. More important, the plant's value in treating dropsy was noted
a few years after Fuchs's account by the Dutch medical biologist Rembert Dodoens,
who wrote that "for those who have water in the belly . . . it draws off the
watery fluid, purifies the choleric fluid, and opens the obstruction."
Digitalis had been included in the London Pharmacopoeia by 1661, though
recommended for the wrong purposes, for epilepsy and sedation. And a few years
later an English physician, William Salmon, became convinced that digitalis was
the long‑sought treatment for tuberculosis.
Salmon's error was a logical one at the time, since there was still confusion
between pulmonary tuberculosis, where digitalis is of no value, and pulmonary
edema or fluid in the lungs due to heart failure, which can be dramatically
helped by digitalis. When an eighteenth‑century physician administered digitalis
to a patient with pulmonary edema thinking the patient had tuberculosis, and the
patient's condition improved, no conclusion could be more obvious than that
here was a remedy for tuberculosis. This mix-up between pulmonary tuberculosis
and pulmonary edema persisted well into the nineteenth century.
No confusion, however, existed in Salmon's mind about the side effects and
toxicity of digitalis when given in excessive amounts. For this reason he
recommended that the drug be given only in very small doses. When doctors
ignored this advice and subsequently killed patients with digitalis, the Dutch
physician Hermann Boerhaave, one of the most respected men in eighteenth‑century
Europe, declared that digitalis was a poison and cautioned against its use.
The most crushing indictment of digitalis came with the experiments of a Dr.
Salerne of Orleans in 1748. Hearing that a turkey had died after eating
foxglove, Salerne proceeded to stuff foxglove powder down the throats of two
healthy turkeys in the vigorous manner that Frenchmen usually reserve for geese
in the making of pâté de foie gras. The two turkeys did not survive these
ministrations, and an autopsy revealed that their intestines had been squeezed
as dry as grapes in a wine press.
Salerne should have concluded that digitalis was indeed a good drug for ridding
the body of excess fluid, but instead be reported to the French Academy of
Sciences that the compound was a powerful poison. The Academy, at that time the
final authority in European medicine, condemned the use of digitalis in medical
practice, and here matters stood for a quarter century until William Withering
decided to reinvestigate the subject.
Withering was born in 1741 into a family of distinguished physicians. His
maternal grandfather had delivered Samuel Johnson, and his father was a
successful physician at Wellington in Shropshire. William was afforded the
impeccable education of the English upper class, which included mathematics, the
classical languages, geography, and history. He was an average student and
showed no sign in school of the remarkable insight that was to result in his
discovery.
At the age of twenty‑one Withering decided to study medicine, and entered the
University of Edinburgh, a school which had appeal for English students in the
eighteenth and nineteenth centuries. Oxford and Cambridge had degenerated into
shadows of their former selves, becoming chiefly schools for the training of
clergymen. The historian Edward Gibbon had left Oxford in disgust after one year
because he found his tutors "plunged deep in port and prejudice." But the
Edinburgh faculty was superb, numbering among its members some of the prominent
scientists of the day.
Withering made many warm friends at Edinburgh, and throughout his life continued
to correspond with teachers and classmates of his student days. He learned to
play golf on the Scottish greens, and also became an accomplished musician with
an incredible mastery of the German flute, the harpsichord, and the bagpipes.
He took his degree of doctor of physic on July 31, 1766, after a successful
defense of an inaugural dissertation on malignant putrid sore throat, later
published under the title De Angina Gangrenosa.
In the eighteenth century no fashionable young English gentleman could consider
his formal education complete until he had made the de rigueur trip to the
Continent. Withering made such a journey during the summer and autumn of 1766
with a companion, a Mr. Townsend, described as "a gentleman of independent
fortune familiar with the manners and language of the French." Midway through
the trip Mr. Townsend had a bit of bad luck, described by Withering in a note to
his parents: "I have been so much taken up for some days past that it was
impossible to find time to write; I have lost my Fellow traveller Mr. Townsend;
an abscess formed upon his shoulder, a Fever came on, the wound gangren'd and
yesterday he died." This was the frequent result of an infection before the days
of antibiotics.
After arriving home at Christmas, Withering began to consider the matter of
setting himself up in a general practice. Flattering offers came from Chester
and Coventry, but he finally selected the small town of Stafford, county seat of
Staffordshire. This was a spot fairly near home, where the medical reputation
both of his father and his uncles was well known. He became physician to the
newly built infirmary, and though he was well liked, the people of the community
were slow to accept a newcomer, leaving free time for the new doctor to fill.
Withering chose to occupy this time engaged in the study of botany, and for a
romantic reason. As a student he had detested the subject, speaking of "the
disagreeable ideas I have formed of the study of botany." But one of his first
Stafford patients was a charming young woman, Helena Cooke, whom it was
necessary to visit almost daily. Miss Cooke passed the hours of her long
convalescence by painting flowers, and Withering fell into the habit of
searching the countryside for new specimens. Her style was so admired that on
September 17, 1772, Miss Cooke became Mrs. Withering.
Along with a new wife, Withering had managed to acquire a first rate knowledge
of plants, and he soon published A Botanical Arrangement of All the Vegetables
Naturally Grown in Great Britain According to the System of the Celebrated
Linnaeus, still considered a classic text. One particular section is of interest
today, because it contains the first cautious comments on the medicinal
properties of the foxglove: "A dram of it taken inwardly excites violent
vomiting. It is certainly a very active medicine and merits more attention than
modern practice bestows on it."
The study of foxglove that made Withering’s reputation was prompted by a medical
consultation: "In the year 1775, my opinion was asked concerning a family
receipt for the cure of the dropsy. I was told that it had long been kept a
secret by an old woman in Shropshire, who had sometimes made cures after the
more regular practitioners had failed. I was informed also, that the effects
produced were violent vomiting and purging; for the diuretic effects seemed to
have been overlooked. The medicine was composed of twenty or more different
herbs; but it was not very difficult for one conversant in these subjects, to
perceive, that the active herb could be no other than the Foxglove."
The diuretic effect referred to is the one so valuable in the treatment of
dropsy, for when it occurs, the body rids itself of copious quantities of
unwanted fluid through the kidneys. Withering noted this beneficial action in a
few patients he treated in Stafford, but presently another opportunity to use
digitalis arose. An offer came from Erasmus Darwin, grandfather of Charles
Darwin, to sponsor a practice in Birmingham, and Withering was soon busier than
ever before with dropsy cases. Here is a record of the remarkable results in
one, a Miss Hill of Aston, who was in the last stages of heart failure with
extreme shortness of breath when a liquid digitalis preparation was administered
by mouth:
"The patient took five . . . draughts, which made her very sick, and acted very
powerfully on the kidneys, for within the first twenty-four hours she made
upwards of eight quarts of water. The sense of fullness and oppression across
her stomach was greatly diminished, her breath was eased, her pulse became more
full and regular, and the swellings of her legs subsided.
"26th. Our patient being thus snatched from impending destruction, Dr. Darwin
proposed to give her a decoction of pareira brava and guaiacum shavings, with
pills of myrrh and white vitriol; and if costive, a pill with calomel and aloes.
To these propositions I gave a ready assent.
"30th. This day Dr. Darwin saw her, and directed a continuation of the medicines
last prescribed."
This case is quite a notorious one in the history of digitalis. Withering,
writing the report nine years later, stated that when he suggested the drug,
"Dr. Darwin very politely acceded immediately to my proposition and, as he had
never seen it given, left the preparation and dose to my direction." Yet Darwin
jumped into print almost immediately after with a paper that did not mention
Withering, and forgot him again in another article, "An Account of the
Successful Use of Foxglove in Some Dropsies and in the Pulmonary Consumption,"
published in the Medical Transactions of the College of Physicians. The two men
then became bitter enemies, though Withering was to retain priority for his
discovery.
Darwin was not able to convince the medical profession with his two
unscrupulously published works that he had found anything worthwhile. Withering
managed to inaugurate the systematic use of digitalis. He kept full records of
his extensive case experience, and after ten years' careful observation
published in 1785 a detailed and systematic treatise, An Account of the
Foxglove, and Some of its Medical Uses: with Practical Remarks on Dropsy and
Other Diseases.
In this book, the fundamentals of digitalis treatment were correctly established
for the first time. A dose of one or two grains twice daily was advised for
patients beginning therapy, the amount still prescribed today. Withering also
recognized that slow digitalization required several days to achieve. Equally
important, he appreciated digitalis toxicity and cautioned against over dosage.
He found that the drug was effective until evidence of its action upon "the
kidneys, the stomach, the pulse, or the bowels" was apparent; then it was to be
stopped. The description of an overdosed patient is most striking.
"I have lately been told that a person in the neighborhood of Warwick possesses a
famous family recipe for the dropsy, in which I Foxglove is the active medicine,
and a lady from the western part Yorkshire assures me that the people in her
country often cure the selves of dropsical complaints by taking Foxglove tea. In
confirmation of this I recollect about two years ago being desired to visit a
traveling Yorkshire tradesman. I found him incessantly vomiting, vision
indistinct, his pulse 40 in a minute. On enquiry it came that his wife had
stewed a large handful of green foxglove leaves in half a pint of water and
given him the liquor which he drank at a draught in order to cure him of an
asthmatic affection. This good woman knew the medicine of her county, but not
the dose of it, for her husband narrowly escaped with his life."
Especially important in the Account was Withering's brilliant recognition of the
action of digitalis, "a power over the motion of the heart, to a degree yet
unobserved in any other medicine." Thus did Harvey's discovery of the
circulation first become applicable to a pathologic condition.
By the time the Account was published, Withering had become highly successful.
His practice had grown to bring him an annual income of two thousand pounds per
year, an immense sum at that time, despite the fact that he held a daily free
clinic for the poor and is said to have treated three thousand cases annually
without charge. He had also become a member of the Lunar Society, a select
scientific organization that numbered among its members James Watt, inventor of
the steam engine, Josiah Wedgewood, the pottery manufacturer, and Joseph
Priestley, the discoverer of oxygen. Benjamin Franklin was a guest at one
meeting, and he consulted Withering by letter regarding the treatment of his
kidney stones.
But just as he reached the peak of his career, Withering was struck by
consumption. Two serious attacks in 1783 and 1786 forced him to give up work
entirely and go to the country to regain his health. In 1790 he had a serious
attack of pleurisy, and until his death he was plagued by shortness of breath
and frequent coughing up of blood. To escape the damp English winters, he spent
two seasons in Portugal, somehow finding strength to study the tropical and
semi-tropical plants not seen in England.
The 1793 trip to Portugal proved to be his last. He bought a beautiful country
estate in September 1799, but on the date he was to move in, Mrs. Withering was
taken ill and could not accompany him; this, in addition to the fatigue of the
journey, sent him to bed, and he died October 6, 1799. During the last days, a
friend who came to see him produced the most distasteful pun in the history of
medicine: "The flower of English medicine is indeed withering."
The Flame of Life
In 1794, five years before William Withering's quiet death in bed, another
scientist had died much more violently, on the guillotine. This man, Antoine
Lavoisier, demonstrated conclusively why breathing is necessary. Lavoisier, a
chemist and not a physician, was able to show that breathing allows the flame of
life to burn in essentially the same way as the flame of a candle, by
combustion of carbon.
Though respiration is one of the oldest physiologic functions man has observed,
for thousands of years it remained the most mysterious. The eyes were necessary
to see, the ears to hear, the kidneys to make urine, the heart to pump; but what
did the lungs do?
The ancient Greeks thought they had the answer: The lungs somehow sucked the
blood from one part of the body to another. Aristotle was sure he had a better
idea. Noting that the lungs were well supplied with blood, he postulated that
their function must be to cool the blood and produce mucus. A few years later,
Erasistratos, who had brilliantly recognized the pumping function of the heart,
stated that the arteries were empty pipes filled with air by the lungs. Galen
rectified this error by demonstrating that the arteries contained blood and not
air, but was unable to explain breathing.
So matters stood for seventeen centuries until an Englishman, Robert Boyle,
began to experiment. Today every high school chemistry student recognizes the
name of this scientist in Boyle's law: The volume of a gas is inversely
proportional to the pressure on it, provided temperature is kept constant. But
Boyle was responsible for much more, including investigation of the physics of
colors, the chemistry of acids and bases, and the specific gravity of body
fluids.
Boyle's work on respiration was stimulated by news of a novel invention. In
1650 a German, Otto von Guericke, had used a suction pump to empty a wine barrel
filled with water and produce what all philosophers had believed to be
impossible-‑a vacuum. Going further, Guericke pumped the air from two metal
hemispheres and then amazed a large group of spectators by connecting a team of
horses to each hemisphere. Straining in opposite directions, the two teams could
not pull the hemispheres apart. Guericke was obviously something of a showman,
since he probably knew that simply anchoring one of the hemispheres to a wall
could have eliminated one of the teams of horses.
When in 1657 Boyle read the first accounts of these experiments he set out to
construct a similar device. The resulting pump designed by his assistant, Robert
Hooke, was easily operated by one man and moderately airtight-‑the first
deliberately designed air pump. Its receiver, seven to eight gallons in volume,
was made of glass and fitted so that objects could be readily put into it before
pumping and then could be manipulated in the vacuum.
With his new air pump, Boyle was able to carry out a large number of
experiments. He demonstrated how a deflated bladder swelled in the vacuum, how
the mercury in a barometer fell, and how the ticking of a watch suspended by a
thread grew fainter and stopped as air was removed. Most dramatic was the way a
bird or kitten without air languished and eventually died in an environment that
would not allow a candle to bum. Throughout the next century this fascination
with the effect on animals ensured that Boyle's experiment was widely repeated
by amateur scientists and is now represented by a painting in the Tate Gallery
in London, "The Air Pump," by Joseph Wright of Derby. In a charming genre scene,
Wright depicted an experimenter using the pump to suffocate a bird in a bell
jar, while a small child looks on in horror.
Robert Hooke, working on his own, went a bit further than Boyle had. Opening
widely the thorax of a dog, he demonstrated that the animal could be kept alive
by artificial respiration in absence of all movements of the chest wall. This
experiment had been done before, and is described in the writings of Vesalius.
But Hooke ingeniously demonstrated that the animal could also be kept alive
without any movement of the lung at all. To do this, the lung was kept
motionless but thoroughly distended by maintaining a powerful blast with a
bellows, the air driven in escaping continually through minute holes pricked in
the lung. Thus the mere movement of the lungs in breathing, previously believed
to be the essential factor, was shown to be unimportant. The purpose of
breathing was to keep a supply of fresh air in constant contact with the lung
tissue.
Hooke is perhaps the most eccentric figure in the history of science. He left
his imprint in chemistry and physics as well as physiology. Physics students
remember him for Hooke's law: The displacement of an elastic body is directly
proportional to the force applied to it. He was also the foremost English
microscopist. But during his lifetime visitors attracted by his fame must have
been surprised when meeting him.
No artist or rock musician of today has a shaggier or more unkempt appearance
than did Hooke. His massive uncombed mane almost covered an ashen face, while
his crooked figure and shrunken limbs grew smaller and more deformed with the
years. His parsimony and his crabbed, sour, jealous, vain, and morbid character
made him one of the most well‑known misers in England.
Not being satisfied to make many of the scientific discoveries of his age, Hooke
also demanded the rest. When Newton's Principia was in the process of
publication, Hooke so insistently claimed part of the work was stolen from him
that Newton determined to suppress a third of the volume, and would have done so
except for the intervention of Edmund Halley. Later, when Newton completed
Optics, he ascertained that Hooke had claims upon it, and steadfastly refused to
publish until after Hooke was dead.
When Hooke died, several thousand pounds were found in an old iron chest in the
scientist’s dingy lodgings; the rusty key was said to have been unused for
thirty years, though Hooke’s physiologic experiments had provided another key
that was employed almost immediately.
In 1669 Richard Lower, an English physician, used Hooke's method of artificial
respiration to observe the blood in the pulmonary veins-‑the vessels that go
from the lungs to the heart. Lower noted that when an animal was suffocated, the
blood in the pulmonary veins and the left side of the heart became dark and
venous. Taking this dark blood and injecting it into the lungs, he found that it
became bright red only if fresh air was driven through the lungs
simultaneously. He concluded correctly that the change in color was due simply
to the exposure of the blood to air in the lungs. This was confirmed by the fact
that a clot of dark venous blood soon became bright red on the upper surface
where it had been exposed to air, and if it was turned upside down, the dark
undersurface also turned bright red.
Following Lower's work, the final important seventeenth‑century observation of
respiration was made by another Englishman, John Mayow. Mayow used a bell jar
inverted over water in which he placed small animals, lighted candles, and
combustible materials, noting that the extinction of life and flame were
associated with a reduction in volume of the contained air. Though thereby
proving that only a portion of the air is necessary to support life and flame,
Mayow failed to identify this portion as oxygen. Here knowledge of respiration
might have remained indefinitely fixed but for the revolution that was taking
place in chemistry.
Chemistry had evolved from the alchemy of the Middle Ages and its preoccupation
with converting metals such as lead into gold. To the alchemists, all matter was
supposed to be made up of a "prima materia" modified by four elements: earth,
air, fire, and water. By the eighteenth century, these had been differentiated
into three varieties-‑mercurial, vitreous, and combustible. In addition to the
elements, there were four spirits-‑sulfur, mercury, arsenic, and Sal ammoniac.
There were also six bodies: gold, silver, copper, lead, tin, and iron. And the
"soul" of all matter was believed to be a hypothetical substance, phlogiston,
by virtue of which all combustible bodies burned.
Joseph Priestley, the son of a weaver in the small English town of Leeds, made
the first large cracks in this imposing alchemical structure. Orphaned at an
early age, Priestley had been adopted by an aunt, a strong‑minded woman of
independent temper, whose influence led to his ordination as a Calvinist
minister who eventually adopted Unitarian views.
On a trip to London shortly after his marriage, Priestley met the philosopher
from the American colonies, Benjamin Franklin, and the encounter was a turning
point. Up to this time, the young minister had taken only a casual interest in
science, but when he suggested to Franklin that someone ought to write a popular
book on electricity, Franklin urged him to do so. The result was Priestley's
brilliant work The History and Present State of Electricity. In writing, he was
led to investigate for himself certain disputed points of electrical theory,
and, through his natural flair for research, he made some original discoveries,
one of which was the fact that carbon is an excellent conductor of electricity.
So successful was the book that a year after its publication Priestley was
elected to the Royal Society.
Priestley's blow to the old alchemical theories came with his discovery that air
is not an elementary substance. Instead, it is composed of several gases, one
of which, oxygen, which he called "dephlogisticated air," is the one essential
to the life of animals. On August 1, 1774, he made some oxygen and was
astonished at how brightly a candle burned in it. On March 8, 1775, he put a
mouse into oxygen and noted how well the animal breathed in it. Shortly
thereafter he wrote to Franklin, "Hitherto only two mice and myself have had the
privilege of breathing it." His further experiments revealed that green plants
breathe out oxygen in sunlight, thus providing for the animals that need it.
Though extremely conservative scientifically, Priestley was quite radical in his
theological and political beliefs, and was as well a rather difficult, cold,
cantankerous, precise, prim, puritanical individual. Naturally, a scientist does
not achieve immortality for his pleasing personality, but in Priestley's case, a
more acceptable disposition and set of political convictions might have made
life much smoother.
Priestley got into trouble with his support of the French Revolution, a very
unpopular issue in some parts of England. In 1791, on the second anniversary of
the fall of the Bastille, he had joined a group of friends to celebrate the
event when a hysterical mob that had set fire to two dissident churches set out
to burn down Priestley's house, hoping to be able to lynch him and his family as
well. Priestley tried to bribe the leaders and, failing, took refuge with
friends while the howling crowd looted his house, scattered his papers, battered
down the walls, and made a bonfire of the debris.
Several hours later the mob went in search of Priestley. He and his family
escaped in a coach with only a few minutes to spare, immigrating to the United
States not long afterward. In America he continued to be hounded by tragedy.
First his favorite son died and, shortly afterward, his wife, who had never
recovered from the shock of the riots. Yet Priestley did become a close friend
of President Thomas Jefferson, who once told him, "Yours is one of the few lives
precious to mankind."
Priestley’s contemporary at the University of Edinburgh, Joseph Black, was a
man able to engage in research unhindered by violent mobs. Studying the
decomposition by heat of caustic lime, Black identified a by‑product, the gas
carbon dioxide, then known as "fixed air." Jean Baptiste van Helmont had
produced this substance a hundred years before, but Black went further by
demonstrating that it was present in the expired air of man and would not
support life.
Now most of the information needed to understand the process of respiration was
at hand. The man who finally provided this understanding, with theory supported
by experiment, was Antoine Laurent Lavoisier.
Some scientists are facile theoreticians but poor experimenters. William Harvey,
who recognized the circulation of the blood though he could not even measure the
pulse rate accurately, was one such. Gregor Mendel, who perceived that
individual hereditary characteristics are determined by two particles, though he
very probably falsified his experimental results to prove this, was another. Two
further examples are James Watson and Francis Crick, who determined the DNA
structure using only chemical theory and the facts about the molecule already
known.
Other scientists are skilled experimenters without being theoreticians.
Rosalind Franklin, who identified and made elegant X‑ray crystallographic
studies of the A and B forms of DNA, is an example. But Lavoisier was both a
superb experimentalist and a brilliant theoretician. His contribution to the
science of healing is universally regarded as the most important of all those
made in the eighteenth century.
Lavoisier was born in Paris on August 26, 1743, the only son of well‑to‑do
parents. His mother died when he was quite young, and his father and a maiden
aunt brought him up with loving care. His father wanted him to become a lawyer,
and Antoine dutifully complied, studying law at Mazarin College, qualifying as
Bachelor of Law in 1763 and as Licentiate in 1764.
A taste for science had developed in the young man. In college he had taken
courses in astronomy, botany, chemistry, and geology, and upon finishing law
school he quickly turned to science again. In 1765 Lavoisier published his first
paper on chemistry, using a careful quantitative approach. He also won a medal
from the king in a prize essay contest on metropolitan street lighting. In 1768,
when he was but twenty‑five years old, he was admitted to membership in the
Academy of Sciences. Soon Lavoisier was being assigned to commissions upon the
recommendation of the eminent scientists in France, usually also writing the
reports of the commissions' findings for presentation to the Academy. But at
just this time he made the mistake that was to cost him his head.
Because of his resolve to pursue a career in science, Lavoisier arranged to
assure himself of sufficient financial means by buying a part share in the Ferme
Générale, a widely hated, privately owned organization to which the French
monarchy farmed out a profitable tax‑gathering monopoly. Throughout his life,
Lavoisier profited by his association with this organization and he seems to
have been quite fair with those from whom the taxes were collected.
At age twenty‑eight, Lavoisier married Marie Anne Pierrette Paulze, the
fourteen‑year‑old daughter of a prominent member of the Ferme. Though it was a
marriage of convenience arranged by the father to save his daughter from
marrying an elderly, disreputable nobleman, Lavoisier and his young bride proved
to be a happy pair. Marie was both a gifted linguist and a skilled artist, who
translated scientific works for her husband and prepared excellent illustrations
of his experiments. As a hostess she made the Lavoisier home a popular meeting
place for French and foreign scientists. And after her husband's execution she
edited and printed privately his last work, compiled in prison, Memoires de
Chimie. Her life was ultimately embittered by an unhappy, short second marriage
to Count Rumford. Rumford was a renowned scientist and inventor, but also an
adventurer possessing no patience with his wife's domestic inclinations. During
one party, to show his disdain for Marie, he publicly threw a pot of boiling
water over her prized bed of flowers. During such times, the former Mme.
Lavoisier no doubt longed for the period during which she assisted her first
husband in his experiments.
Of these, the most important are the ones Lavoisier performed at age
twenty‑nine, one year after his marriage. While they were in part a repetition
of Priestley's work, both men were actually performing almost a caricature of
one of the classic experiments of alchemy.
First, red oxide of mercury was heated using a burning glass, in a vessel in
which the gas-produced‑oxygen could be observed and collected. This was the
qualitative part of the experiment, but to Lavoisier's mind a revolutionary new
idea was suggested: Run the experiment in both directions and measure exactly
the quantities that are exchanged. Initially, mercury was burned so that it
absorbed oxygen, and the exact amount of oxygen taken up from a closed vessel
was determined from the difference in weight between the beginning of the
burning and the end. Then the process was reversed by vigorously heating the
mercuric oxide formed so that it would again expel the oxygen. When elemental
mercury was left behind and the oxygen had flowed into the vessel, Lavoisier
once more measured the proportions and found exactly the same amount of oxygen
was given of as had been taken up before.
Quite suddenly, the process of chemical combination had been revealed for what
it is, the coupling and uncoupling occurring between fixed quantities of two
substances. Lavoisier had brilliantly dispatched the old notions of phlogiston,
essences, and principles by showing that two elements, mercury and oxygen, had
been demonstrably put together and taken apart. As he said, "This discovery,
which I have established by experiments that I regard as decisive, has led me to
think that what is observed . . . may well take place in the case of all
substances that gain weight by combustion. . . ."
These new principles were slowly accepted by most scientists, though a few
continued to cling to the old theory. A whole new nomenclature had to be
devised, for "earth," "air," "fire," and “water" were no longer sufficient.
Lavoisier, with other leading French chemists, composed this terminology, and
with minor revision it is still in use today.
Early during his studies of combustion Lavoisier guessed that what had occurred
in his glass beakers might also occur in the animal body. Working with the
French mathematician Pierre Laplace, he designed a set of elegant experiments
to verify this theory. By accurately measuring a guinea pig's intake of oxygen
and output of carbon dioxide and heat-‑the latter with an ice calorimeter they
invented-‑the two men were able to demonstrate that the animal produced the same
amount of heat during consumption of a predetermined amount of oxygen as was
produced by burning charcoal consuming the same amount of oxygen. From their
studies, Lavoisier and Laplace concluded: "Respiration is therefore a
combustion, admittedly very slow, but otherwise exactly similar to that of
charcoal; it takes place in the interior of the lungs, without the evolution of
light, since the matter of fire set free is immediately absorbed by the moisture
of those organs. . . ."
Lavoisier erred in believing that combustion took place in the lungs rather than
in the tissues, but this mistake was made up for by the breadth and depth of his
other investigations. He was the first scientist to show that water is made of
hydrogen and oxygen and is, therefore, a compound rather than an
element‑-another blow to alchemy. He also made valuable contributions to the
study of political economy, the science of agriculture, and public education.
There is no way to guess what else this brilliant man might have achieved had
he lived longer, for the most violent segment of the French Revolution
tragically coincided with the peak of his scientific career.
In 1793, to repress all counterrevolution, the revolutionary government of
France, called the Convention, created a kind of supreme political police and
set up what we now call "the Terror." Designed to protect the new republic from
its internal enemies, the Terror struck at those who were in league against the
republic, and at those who were merely suspected of hostile activities. The
number of persons who lost their lives in the Terror, from the late summer of
1793 to July 1794, is small by twentieth‑century standards. Yet about forty
thousand persons died in it, most on the guillotine, though some by other
methods. At Nantes, for example, two thousand people were loaded on barges and
deliberately drowned. Victims of the Terror included Marie Antoinette and other
royalists, former revolutionaries, and Lavoisier.
The tax‑collecting Ferme Générale was, after the royal family, one of the first
targets. No one then had any more love for revenue agents than we do today. On
November 14, 1793, Lavoisier, his father‑in-law, and their colleagues on the
Ferme were ordered arrested. Since he had been scrupulously honest in his
dealings, Lavoisier was accused of such crimes as trying to poison the Paris air
by erecting a large wall in the city. When his attorney asked that consideration
be given to Lavoisier's scientific achievements and their benefit to the nation,
the judge, one Jean Baptiste Coffinhal, snapped back that the republic had no
need for scientists.
Lavoisier, dignified and aristocratic to the end, was executed on May 8, 1794,
for "plotting against the people of France." Shortly before he went to the
guillotine, he calmly remarked, "This probably saves me from the inconveniences
of old age."
His body was dumped, along with dozens of others, into an unmarked mass grave.
[1] Chinese physicians also recognized that Digitalis purpurea could be used to
treat tumors. Modern studies have demonstrated that digoxin and digitoxin are of
value in the treatment of brain, blood, breast, and prostate cancer and work by
causing apoptosis, that is, cell death by self destruction. (Haux J. Digitoxin
is a potential anticancer agent for several types of cancer. Medical Hypotheses
53:543-548, 1999)
|